
Vianney Taquet
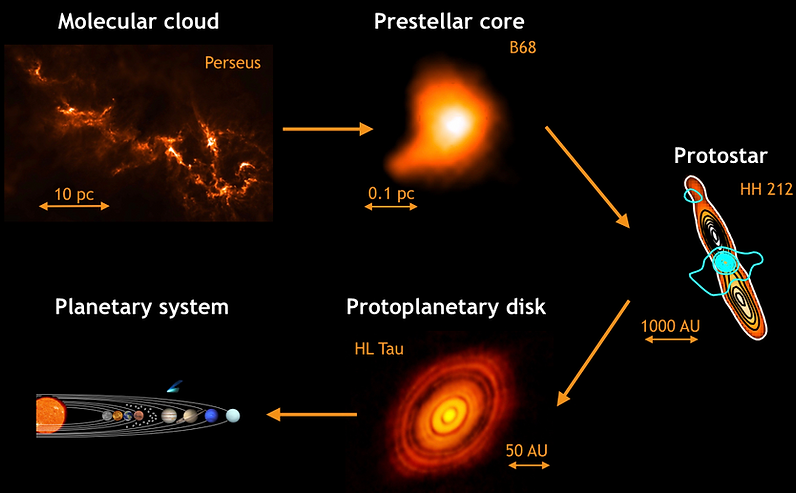
This scheme represents the different stages of star formation, from the Perseus molecular cloud as observed by the Herschel Space Observatory (left) to a planetary system such as our own Solar System. The B68 prestellar core has been observed with Herschel, while the young Class 0 protostar HH212 and the protoplanetary disk HL Tau were observed with the ALMA interferometer by Codella et al. (2014) and the ALMA partnership (2015).
The chemical origin of our Solar System
​
The importance of comets, meteorites, and other small bodies of the Solar System for the origin of organic molecules that triggered the pre-biotic chemistry on Earth is still a matter of debate. Ground observations as well as recent measurements by the Rosetta probe showed that comets are chemically rich, with the detection of many organic molecules such as the simplest amino acid, glycine (Goesmann et al., 2015 , Altwegg et al., 2016). The in-situ analysis of the organic materials present in a specific type of meteorites, the carbon chondrites, revealed the presence of numerous amino and hydroxylated acids (Pizzarello et al., 2006). Most of the cometary and meteoritic materials have remained pristine since the end of the solar nebula. The analysis of their chemical composition could therefore give us some constraints on the formation and evolution of the Solar System.
​
Solar System objects were formed during the protoplanetary phase through the coagulation of interstellar grains. It is at the surface of these grains that the first mantles of volatile ices form in cold dark clouds. These ices are mainly composed of water, carbon dioxide, or simple organic molecules such as methanol. These ice evaporate subsequently in the warm gas surrounding the young protostars, as evidenced by the detection of several tens of molecules in the gas phase of star-forming regions. Several chemical mechanisms in aqueous solution have been proposed to explain the structural and isotopic characteristics of meteoritic molecules. Some mechanisms invoke abundant interstellar species, such as aldehydes, ketones or simple nitrogen molecules, such as ammonia or hydrogen cyanide. Understanding the early stages of stellar formation is therefore crucial to constrain the chemical origin of comets and meteorites. However, despite recent progress, the mechanisms responsible for the molecular evolution during the formation of Sun-like stars remain uncertain, and their importance for the chemistry occurring in the solar nebula must be confirmed.
​
My researchs aim at constraining the physical and chemical processes responsible for the molecular richness observed in star-forming regions and to assess whether this interstellar chemistry can survive until the formation of planetary systems.
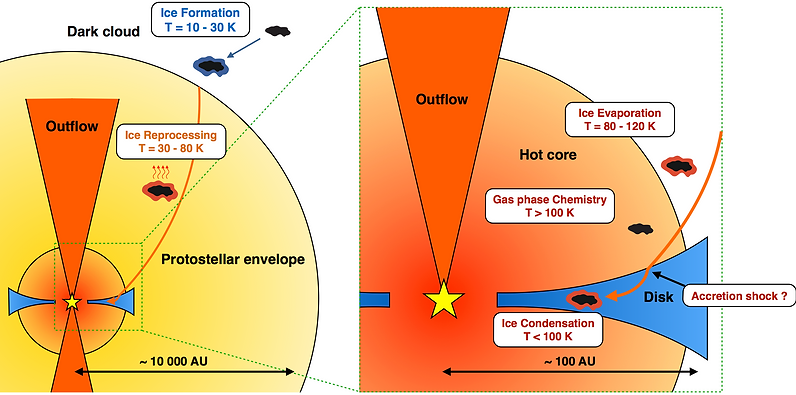
Research highlights​
Linking interstellar and cometary O2: a deep search for 16O18O in the solar-type protostar IRAS 16293--2422
V. Taquet, E. F. van Dishoeck, M. Swayne, J. K. Jørgensen, D. Harsono al. 2018, A&A, in press
​
Recent measurements carried out at comet 67P/C-G with the Rosetta probe revealed that molecular oxygen, O2, is the fourth most abundant molecule in comets. Models show that O2 is likely of primordial nature, coming from the interstellar cloud from which our Solar System was formed. However, gaseous O2 is an elusive molecule in the interstellar medium with only one detection towards quiescent molecular clouds, in the rho Oph A core. We perform a deep search for molecular oxygen, through the 2,1 - 0,1 rotational transition at 234 GHz of its 16O18O isotopologue, towards the warm compact gas surrounding the nearby Class 0 protostar IRAS 16293--2422 B with the ALMA interferometer. The targeted 16O18O transition is surrounded by two brighter transitions at +- 1 km/s relative to the expected 16O18O transition frequency. After subtraction of these two transitions, residual emission at a 3 sigma level remains, but with a velocity offset of 0.3 - 0.5 km/s relative to the source velocity, rendering the detection "tentative". We derive the O2 column density for two excitation temperatures Tex of 125 and 300 K, as indicated by other molecules, in order to compare the O2 abundance between IRAS16293 and comet 67P/C-G. Assuming that 16O18O is not detected and using methanol CH3OH as a reference species, we obtain a [O2]/[CH3OH] abundance ratio lower than 2 - 5, depending on the assumed Tex, a three to four times lower abundance than the [O2]/[CH3OH] ratio of 5-15 found in comet 67P/C-G. Such a low O2 abundance could be explained by the lower temperature of the dense cloud precursor of IRAS16293 with respect to the one at the origin of our Solar System that prevented an efficient formation of O2 in interstellar ices.
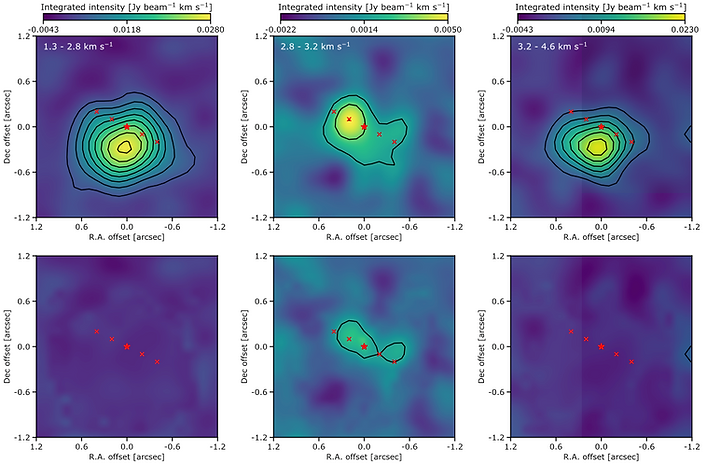
Top: Integrated intensity maps around the 16O18O transition at 233.946098 GHz for velocities of 1.3 − 2.8 (left), 2.8 − 3.2 (middle), and 3.2 − 4.6 (right) km/s. Bottom: Residual maps of the integrated intensity emission after subtraction of the fit performed with the line profile of the reference transition. Contours are in steps of 3σ, with σ of 1.07, 0.54, and 1.08 mJy beam^−1 km/s, respectively. The red star symbols depicts the position of the IRAS16293 B continuum peak while red crosses show the positions of the half-beam and full-beam offset positions mentioned in the text.
A primordial origin for molecular oxygen in comets: a chemical kinetics study of the formation and survival of O2 ice from clouds to discs
V. Taquet, K. Furuya, C. Walsh, E. F. van Dishoeck 2016, MNRAS, 462, 99
​
Molecular oxygen has been confirmed as the fourth most abundant molecule in cometary material (O2/H2O about 4 %) and is thought to have a primordial nature, I.e. coming from the interstellar cloud from which our Solar system was formed. However, interstellar O2 gas is notoriously difficult to detect and has only been observed in one potential precursor of a solar-like system. Here, the chemical and physical origin of O2 in comets is investigated using sophisticated astrochemical models. Three origins are considered: (I) in dark clouds; (II) during forming protostellar discs; and (III) during luminosity outbursts in discs. The dark cloud models show that reproduction of the observed abundance of O2 and related species in comet 67P/C-G requires a low H/O ratio facilitated by a high total density (≥ 105 cm-3), and a moderate cosmic ray ionization rate (≤ 10-16 s-1) while a temperature of 20 K, slightly higher than the typical temperatures found in dark clouds, also enhances the production of O2. Disc models show that O2 can only be formed in the gas phase in intermediate disc layers, and cannot explain the strong correlation between O2 and H2O in comet 67P/C-G together with the weak correlation between other volatiles and H2O. However, primordial O2 ice can survive transport into the comet-forming regions of discs. Taken together, these models favour a dark cloud (or 'primordial') origin for O2 in comets, albeit for dark clouds which are warmer and denser than those usually considered as Solar system progenitors.

Two schemes summarising the proposed chemical scenarios to explain the high abundance of O2 in comet 67P/C-G and the strong correlation with the water signals observed by Rosetta. High abundance of molecular oxygen ice, trapped inside a water ice matrix, can be formed in a dense (nH = 1e5 - 1e6 cm-3) and "warm" (T = 20 - 25 K) molecular cloud, explaining the signals measured in comet 67P/C-G observed by Rosetta. The second scenario, O2 production during the formation of the circumstellar disk, does not allow us to explain the high abundance of O2 and the strong correlation between O2 and water signals observed by Rosetta.
Formation and recondensation of Complex Organic Molecules during protostellar luminosity outbursts
V. Taquet, E. Wirstrom, S. B. Charnley 2016, ApJ, 821, 46
​
During the formation of stars, the accretion of surrounding material toward the central object is thought to undergo strong luminosity outbursts followed by long periods of relative quiescence, even at the early stages of star formation when the protostar is still embedded in a large envelope. We investigated the gas-phase formation and recondensation of the complex organic molecules (COMs) di-methyl ether and methyl formate, induced by sudden ice evaporation processes occurring during luminosity outbursts of different amplitudes in protostellar envelopes. For this purpose, we updated a gas-phase chemical network forming COMs in which ammonia plays a key role. The model calculations presented here demonstrate that ion-molecule reactions alone could account for the observed presence of di-methyl ether and methyl formate in a large fraction of protostellar cores without recourse to grain-surface chemistry, although they depend on uncertain ice abundances and gas-phase reaction branching ratios. In spite of the short outburst timescales of about 100 years, abundance ratios of the considered species higher than 10% with respect to methanol are predicted during outbursts due to their low binding energies relative to water and methanol which delay their recondensation during cooling. Although the current luminosity of most embedded protostars would be too low to produce complex organics in the hot-core regions that are observable with current sub-millimetric interferometers, previous luminosity outburst events would induce the formation of COMs in extended regions of protostellar envelopes with sizes increasing by up to one order of magnitude.
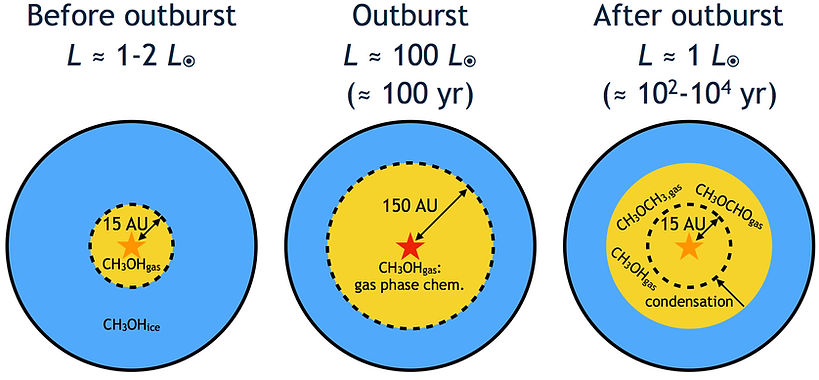